Stalagmites can offer a wealth of information about past changes in environment and climate. Stalagmites are a type of speleothem (cave formation) that grow from water rich in dissolved calcium carbonate (CaCO3) dripping from a cave ceiling onto the cave floor. If conditions are right, the water hitting the ground will leave behind a very thin layer of solid CaCO3 mineral. Over time, this mineral will build up into the formation we call a stalagmite.
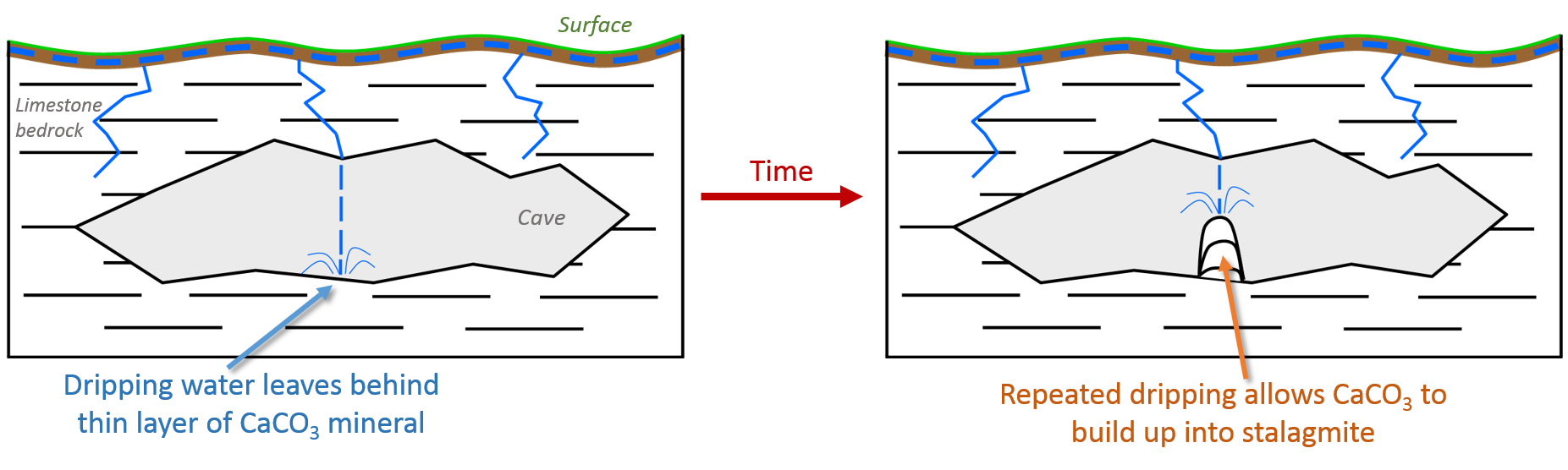
The water that drips in the cave originally comes from precipitation that falls on the surface above the cave. Different weather patterns can change the water isotopic ratios of precipitation, and the meteorological conditions and history of a precipitation event will leave a distinct isotopic signal in the precipitation. As precipitation filters through soil, it can also pick up a carbon isotopic value that reflects the extent and composition of vegetation. Other conditions, such as the temperature and carbon dioxide concentration of the cave, also can leave detectable traces in the isotopes of chemicals contained in the drip water. By studying modern day variability in drip water chemistry, we can link isotopic changes to environmental changes.
If the precipitation that falls on the surface of the Earth eventually makes its way into the cave and drips to form a mineral layer on a stalagmite, this new mineral layer will preserve the isotopic signal of the carbon and oxygen present in the drip water that reflects the current environment conditions above the cave. If the climate or vegetation changes, the isotopic values will change, and new mineral layers in the stalagmite will reflect that change. By analyzing the isotopes of a stalagmite layer by layer and ‘translating’ the stalagmite isotopes into environmental changes, we can reveal long-term trends and shifts in the local climate and precipitation. We call these isotopes climate proxies, and this approach of applying modern understanding of climate-geochemistry interactions to proxy data pulled from the past is the basis for paleoenvironmental studies.
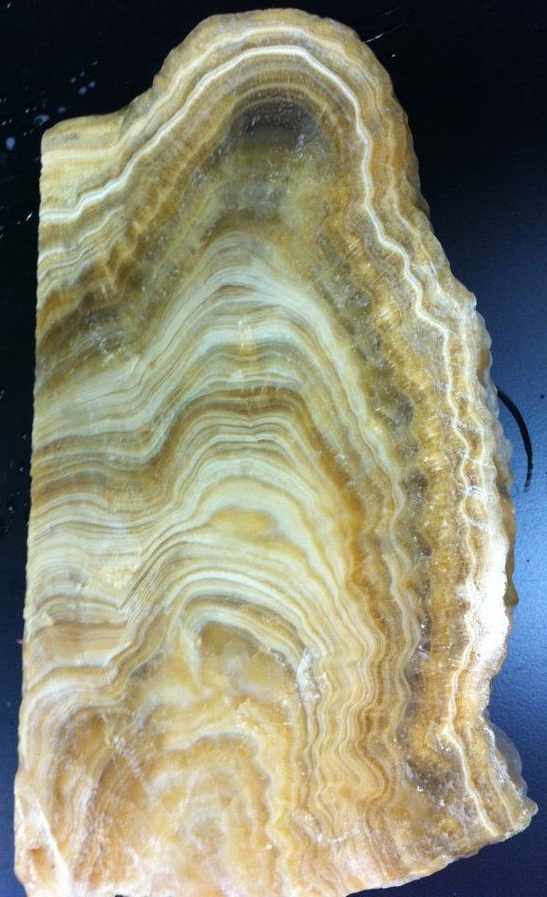
In the laboratory, small (60-90 μg) samples of the stalagmite are drilled in progression along the central growth axis of the stalagmite and analyzed with mass spectrometry to determine the oxygen and carbon stable isotope ratios of each sample. When connected with a dated chronology, the isotope ratios will reveal how the environment changed over the time that the stalagmite grew.
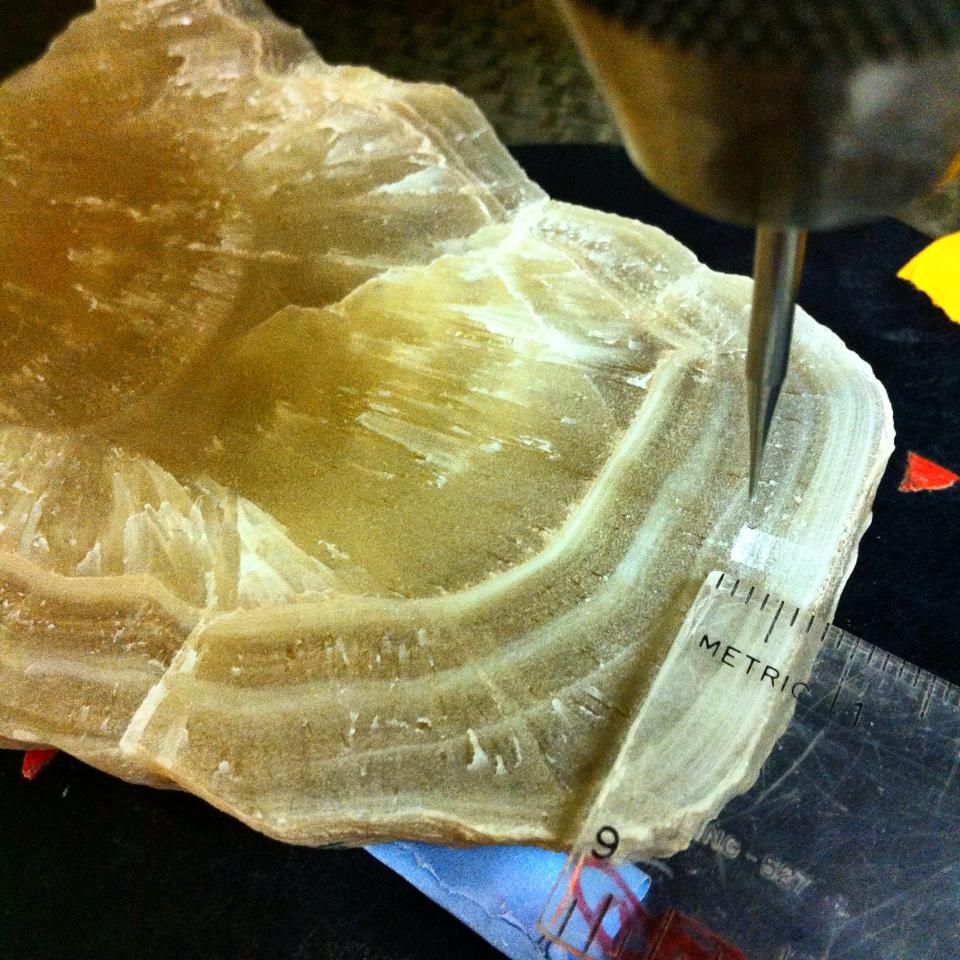
Soils will also leech organic acids into water that later drips on stalagmites and gets locked in the crystal structure. These acids fluoresce under ultraviolet light and can be used as a proxy for soil productivity (since a more productive soil = more organic acids = greater fluorescence). When the stalagmite is cut in half and exposed to ultraviolet light, the organic acids will fluoresce and can be captured in an ultraviolet-stimulated luminescence (UVL) image.
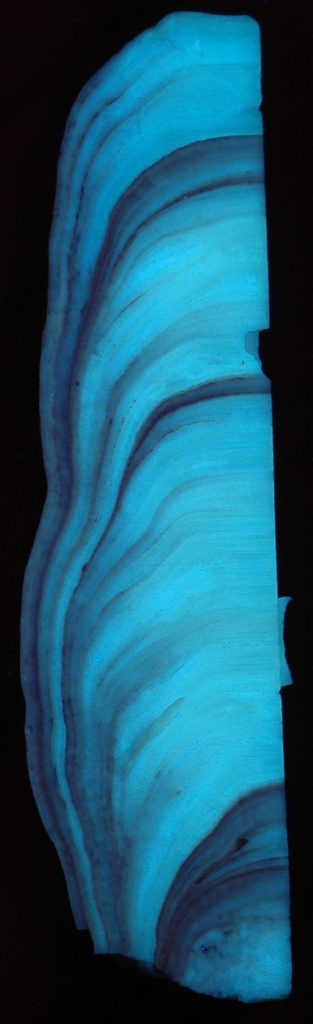
Another lesser-used but valuable climate proxy is petrography, where changes in the mineral crystal structure of the stalagmite is examined under a microscope. Stalagmites can stop growing due to conditions being either too dry OR too wet, but these opposing conditions often produce distinctive patterns in a stalagmite’s structure that are revealed by petrographic analysis. These identifications are critical to accurately creating chronologies for stalagmites.
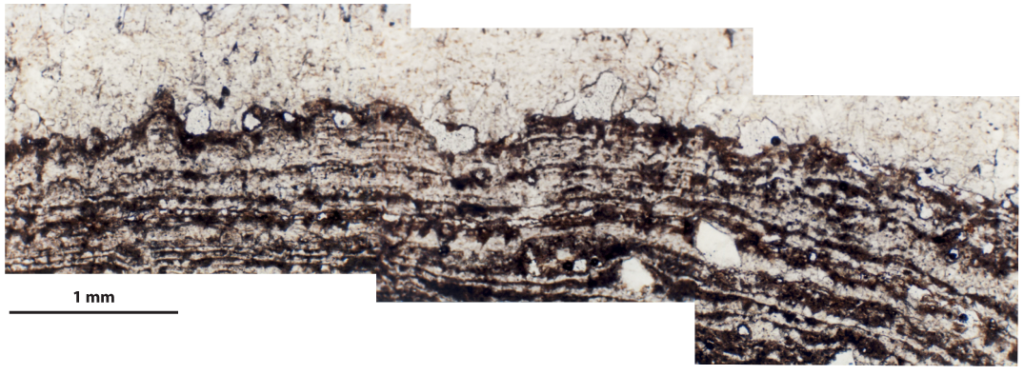
By combining and comparing the environmental stories suggested by each proxy, the overall narrative that emerges (known as a multi-proxy record) often offers a stronger case and more holistic view of environmental changes than a single proxy record alone.
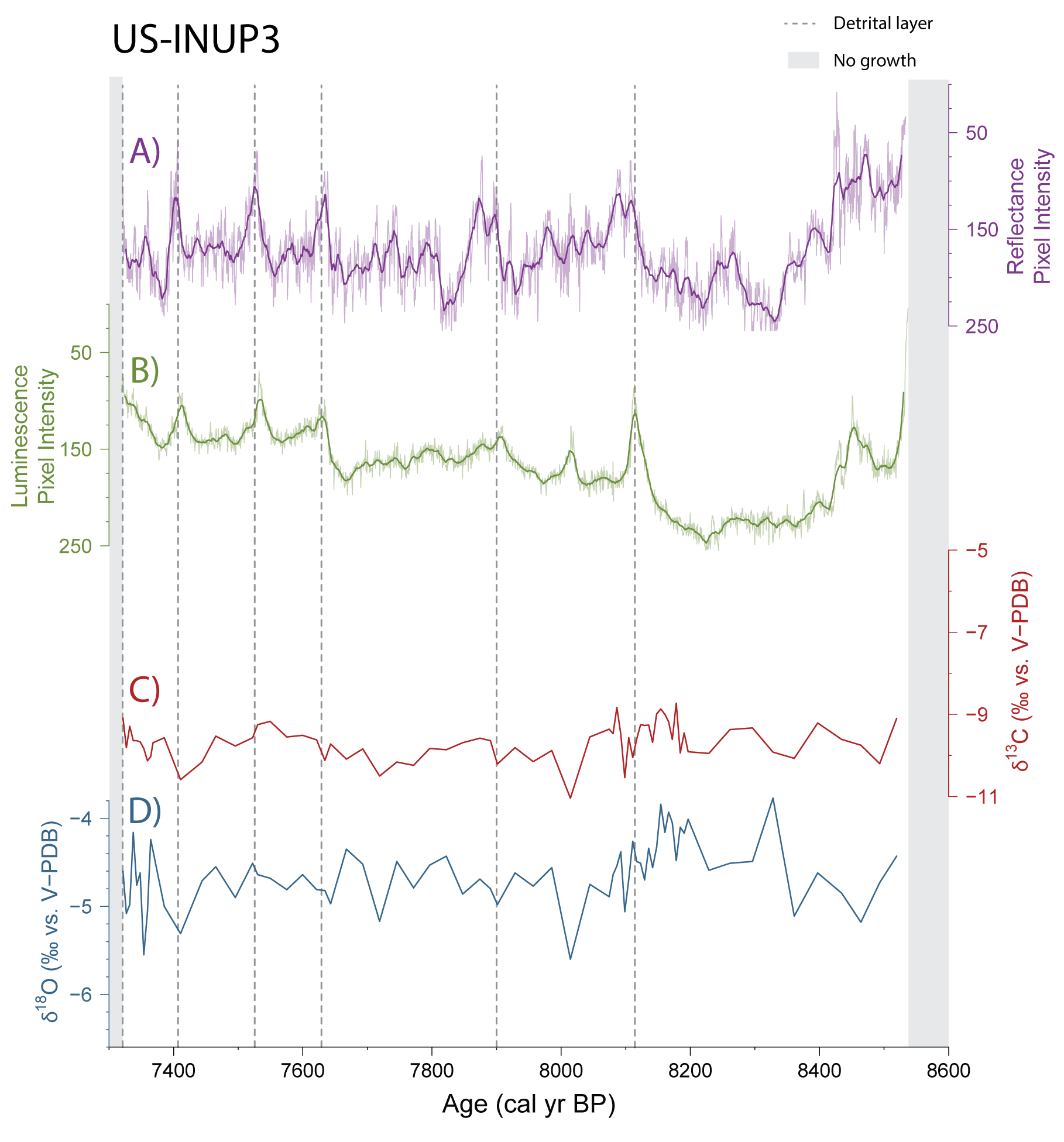