From 2021 to 2022, I worked on Project SCADI (Snow Core Accumulation from Delta-15N Isotopes) as a Marie Skłodowska Curie research fellow, funded by the European Commission as part of its Horizon 2020 funding program. The overall goal of SCADI was to develop a new method of inferring the snow accumulation at a site in Antarctica based on the isotopic ratios of nitrate present in the snow. This required taking snow samples at dozens of sites across East Antarctica and processing the snow to extract, concentrate, and analyze the nitrate, which is naturally present at levels only between ~5 and 500 parts per million in Antarctic snow and ice. By combining real world observations of nitrate isotopes with theoretical models of how exactly sunlight breaking down nitrate changes its isotopes in a predictable manner, we successfully produced a linear relationship linking nitrate isotope values to snow accumulation rates. This newly defined relationship can now be applied to nitrate isotopes preserved in long ice cores to calculate how snow accumulation has changed over time in Antarctica.
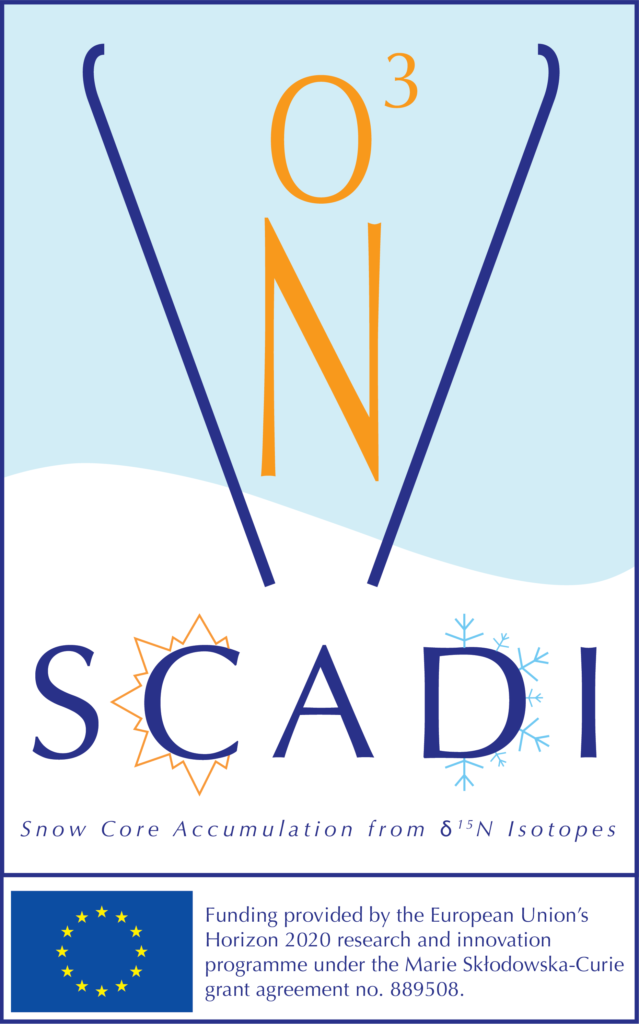
But why do we care about past Antarctic snow accumulation? SCADI follows a classic paleoclimate motivation in that we want to know more about how an environmental system (here, the Antarctic ice sheet) changed in the past so that we can better predict how it will change in the future. The massive ice sheet over East Antarctica is over 4500 m (15000 ft) thick in places and holds enough ice to raise sea levels over 50 m (150 ft) if completely melted. While this ice storage has been stable for the past few thousand years, scientists are very interested in how the glacial mass balance of East Antarctica might change in a future warmer climate. Glacial mass balance describes if a ice sheet is gaining mass (i.e., ice), losing mass, or is stable over a period of time by comparing how much mass is added by snow falling on the ice sheet versus the mass lost by icebergs calving into the ocean at the coasts and surface melting (although East Antarctica is so cold that surface melting almost never occurs). If more mass is added than lost (i.e., it snows faster than icebergs can break away), then the ice sheet as a whole has a positive mass balance and it grows. If more mass is lost than added, the ice sheet has a negative balance and will shrink. A shrinking ice sheet means that less water is being stored as ice on land, and instead more water is being stored in the ocean. As a result, global sea level will rise.
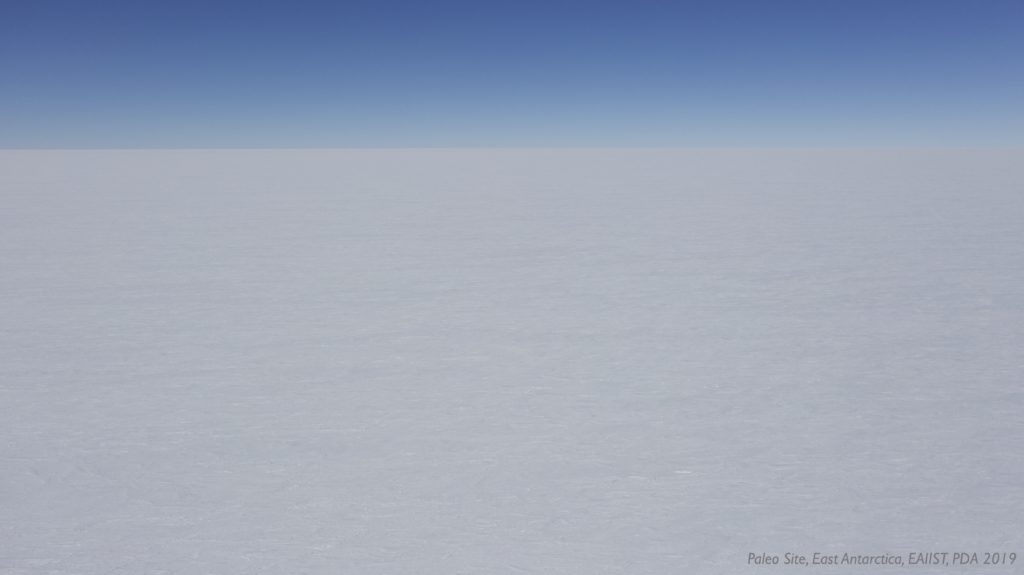
Even though changes to the East Antarctic ice sheet can have dramatic global impacts, we are not certain what the modern mass balance of East Antarctica is or if it is changing to be more positive or negative. While you might expect that warmer temperatures would simply lead to greater ice loss and higher sea levels, warmer air also brings more moisture and snowfall to Antarctica, and this increased snowfall could potentially offset some or all of any increase in ice loss. Observing how the mass balance changed in the past could help us better predict the future, but unfortunately it is nearly impossible to have long-term snowfall monitoring stations across East Antarctica due to its vast and remote expanses.
To make a strong connection between nitrate isotopes and snow accumulation, we need a big dataset that has nitrate samples coming from many sites with a wide range of snow rates. We built this dataset by taking snow samples across East Antarctica that have different snow accumulation rates today and analyzing the nitrate at those sites. While many samples can be taken at existing research stations (such as Dumont d’Urville and Concordia) and along the supply routes that connect these stations, a full dataset requires samples from sites with extremely low snowfall to mimic conditions during the last glacial maximum. To build this dataset, I worked as a member of the East Antarctic International Ice Sheet Traverse (EAIIST).
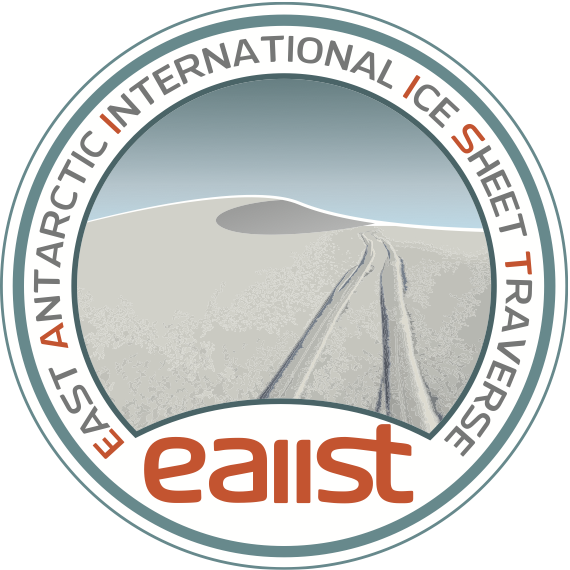
EAIIST was a scientific research caravan that traveled 3600 km across East Antarctica during December 2019 and January 2020. Starting from the Adélie coast, the caravan traveled far inland onto the ultra-dry East Antarctic plateau. After a stop at Concordia Station on the summit of Dome C (>3000 m elevation and home to the 800,000 year long EPICA ice core), the caravan continued toward the South Pole to reach a remote Megadunes site, which today may be a similar environment to what Dome C and other deep ice core drilling sites were like during glacial maxima in the Pleistocene. All along the way, the EAIIST team studied a diverse range of environmental topics, including the weather, snow characteristics and chemistry, ice sheet inner structure, and seismology. On this trip, I took dozens of snow and ice samples to analyze for nitrate isotopes and build up the spatial coverage of our database.
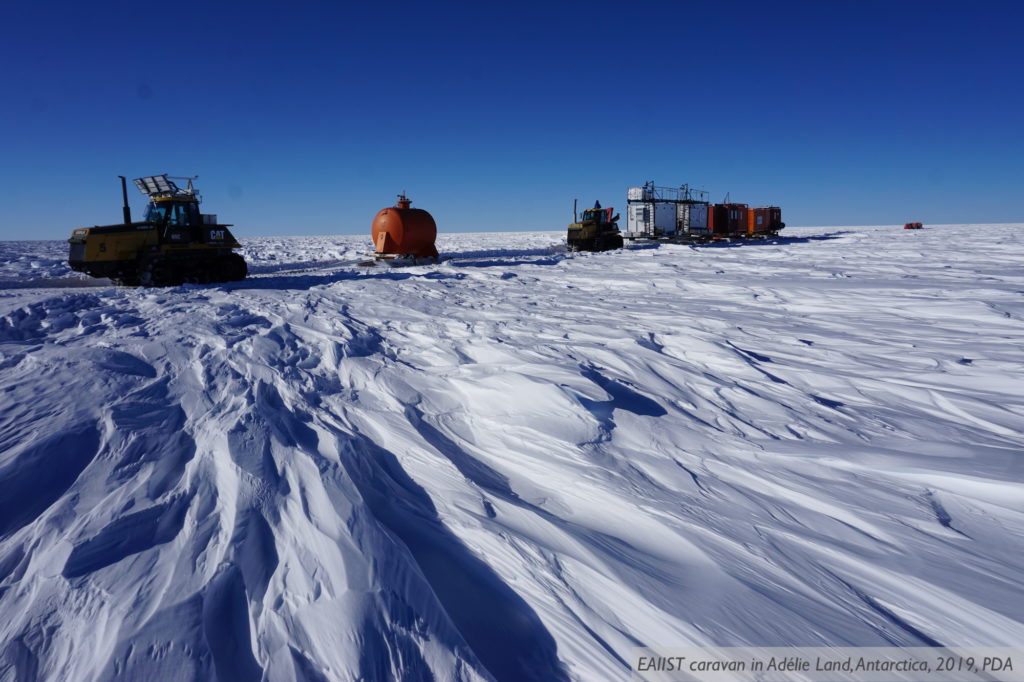
So how exactly can nitrate give us a window into how the weather changed in Antarctica hundreds or even thousands of years ago? Nitrogen has two stable isotopes: the common 14N with 7 protons and 7 neutrons and the rarer 15N, which has an extra neutron. In nitrate (or any compound that contains nitrogen), the nitrogen atom can be either 14N or 15N, and any amount of nitrate will be a mix of both 14NO3– and 15NO3–. We call the ratio of 15N to 14N in a compound ‘delta-15-N’ (δ15N), and this δ15N can be very useful to track environmental changes.
Nitrate naturally falls onto the surface of Antarctica and is eventually buried by later snow and preserved in the ice sheet. During the window of time between deposition and deep burial, some of the nitrate will be struck by ultraviolet (UV) light and converted into nitrogen oxide in a process called photolysis (after photo = light and lysis = break). Nitrogen oxides are gases and can escape from the snow into the atmosphere, leading to an ongoing loss of nitrate in the snow.
Because of its extra neutron, 15N is slightly heavier than 14N. When UV light hits nitrate with a 15N, this extra mass makes it slightly less likely to react and escape into the atmosphere than nitrate with 14N. When this occurs, the δ15N of the remaining nitrate in the snow increases because photolysis is removing nitrate with 14N faster than nitrate with 15N. The longer that photolysis occurs, the greater the increase in δ15N.
Photolysis ends when enough snow falls to bury the nitrate below the depth that light can reach. Therefore, if the rate of snow accumulation is higher (i.e., it is snowing more), the nitrate will be buried faster and the duration of photolysis will be shorter than if it snows less. This means that the δ15N of nitrate is lower when snow accumulation is higher, and this δ15N signal is preserved indefinitely once the nitrate is buried below the reach of sunlight. If we take a a core of ice from a location and measure the δ15N from the bottom to the top, we can use the δ15N to reconstruct the changes in snow accumulation that has occurred at that site.
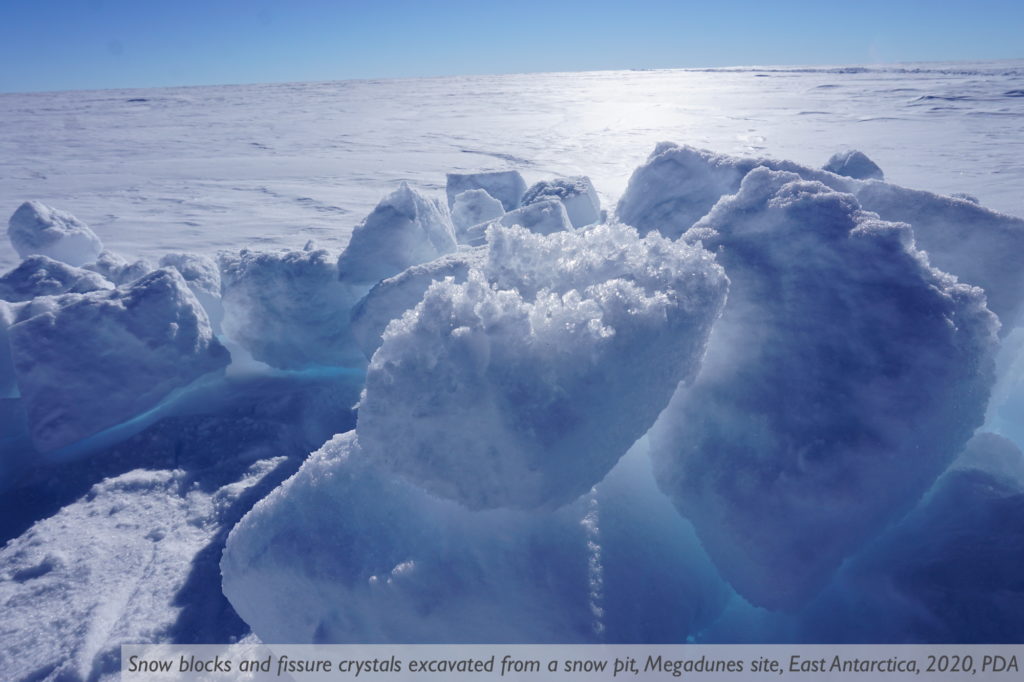
After combining the new data from EAIIST with previously published data, I produced a database with 135 standardized entries of δ15N values paired with local snow accumulation rate. In our 2022 Nature Communications paper, my co-authors and I use this database to define an empirical model that can translate δ15N values into snow accumulation rate. To validate our new technique, we applied our model to an ice core with nitrate isotope data that goes back 700 years. The snow accumulation changes that we reconstructed from the nitrate match very well with changes that we can estimate from the ice core’s density. Our δ15N-accumulation database and the reconstructed ice core accumulation record are publicly available on the PANGAEA data hosting site, and we have made our code (in R) freely available as well. Additional manuscripts focusing on specific regional subsets of our database and its application to other ice cores are ongoing and will be submitted to open-source journals in the coming months.
My research experiences with SCADI and EAIIST are documented in a series of nine weather blog entries. Check them all out in the links here:
Article 1 : Exploring East Antarctica and its role in climate: a first-hand report
Article 2 : A frozen realm: inside the East Antarctic Ice Sheet
Article 3 : Arrival in Antarctica: not your everyday trip
Article 4 : A taste of the traverse: how to cross Antarctica without a plane
Article 5 : Life and work on the frozen continent: Antarctic research stations
Article 6 : Snow, stars, and stress: science at Concordia station
Article 7: Down in the snow trenches: a closer look at an Antarctic research project
Article 8 : Where none have stood before: a return to the EAIIST traverse
Article 9 : Five days of work for 5000 years of history: a closer look at shallow ice core drilling